Hercules Global Solutions
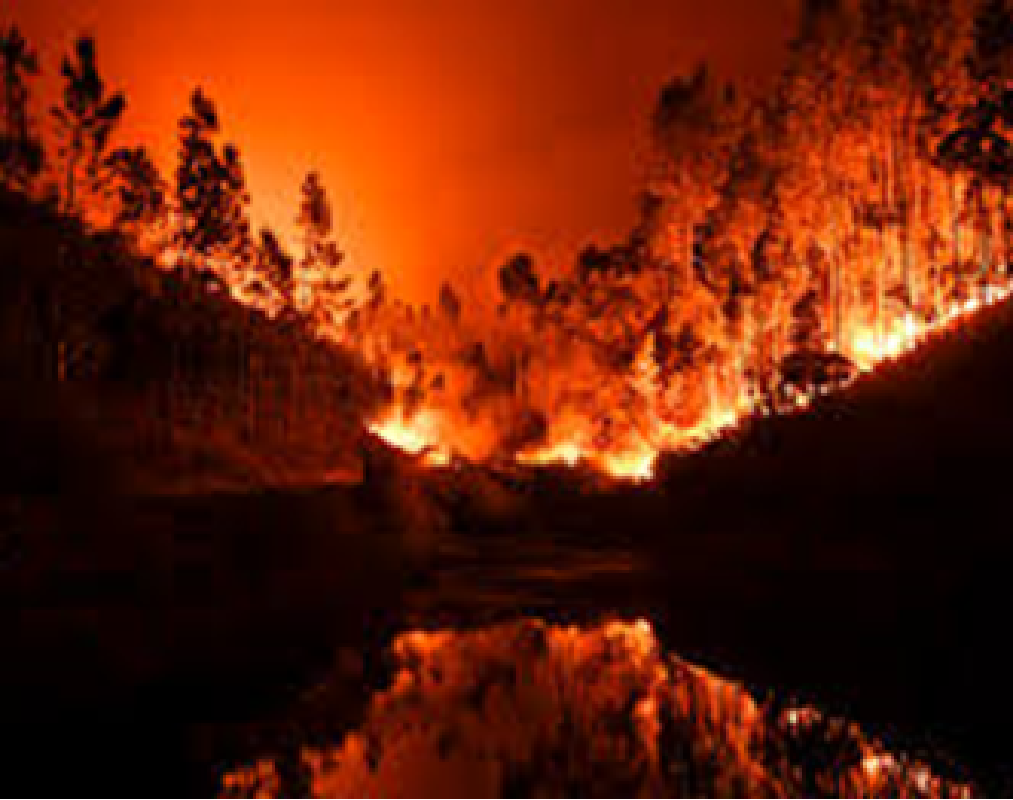
The last seven years were the world’s hottest years on record since record-keeping began in 1880
Unless drastic action is taken soon the world will increasingly suffer from more intense storms, fires, heatwaves, famines, and floods
Banking giant Citigroup estimates that unchecked climate change will cost $44 Trillion by 2060
This presentation discusses the biggest problems related to global warming and suggests unconventional solutions to address them.
The goal of this presentation is to provide a roadmap for the world to significantly reduce global warming.
Table of Contents
Problem: Net zero
Solution: Real Zero
Problem: Over optimistic onshore wind and hydroelectric power
Solution: Climate change adjustments to projected capacity factors
Problem: Carbon offsets
Solution: Continue planting trees but don’t grant carbon offsets for such efforts.
Problem: Traditional QE and Green QE
Solution: Environmental Easing
Problem: Fossil Peaker Power Plants
Solution: Iron-air batteries and Energy Vault gravity-based storage
Problem: Green hydrogen as fuel for cars and heating.
Solution: Closed loop hydrogen and ammonia for long duration and seasonal energy storage.
Problem: Rooftop solar
Solution: Utility-scale solar
Problem: Silicon Based Photovoltaic (PV) cells
Solution: CZTS thin film solar
Problem: COP 26 Methane pledge
Solution: Cut methane emissions by 75%
Problem: Climate change adaptation
Solution: Environmental Easing can be a lifeline for smaller countries
Conclusion
References
Novel Solutions
Problem: Net zero
Solution: Real Zero
As a new Oxfam report stated, ‘Net zero’ carbon targets are dangerous distractions from the priority of cutting emissions. Rich nations and corporates are continuing “dirty business as usual”. Most of their pledges are vague and not backed by measurable plans.
Removing emissions is not a substitute for cutting emissions, and these should be counted separately.
As it is most countries cannot be trusted to follow through on their promises. As the Washington Post recently reported many countries under report their greenhouse gas emissions to the United Nations, in aggregate by anywhere from 8.5 to 13.3 billion tons per year.
Rather than celebrating and letting a country off the hook whenever they say the magical words ‘net zero’ and some far-off date in the future when they will achieve it, it would be helpful instead to assess each country’s commitments and actions and grade them from A to F on how much they are actually decarbonizing.
A simple decarbonization table for each country would show each sector of its economy, what emissions were, what they should be, and how it plans to decarbonize further.
Going forward the goal should be ‘Real zero’ instead of ‘Net zero’. Real zero means zero emissions of carbon. Only with this as the goal will the world be on a credible path to Net Zero. Fanciful plans for carbon removal, such as with Direct Air Capture (DAC) should not allow countries to skip the hard work of decarbonizing.
For an 83% chance of limiting global warming to 1.5 C the world can only emit another 300 Gt CO2 (Gigatons, i.e. billion tons). In 2021 alone the world emitted over 36 billion tons of CO2. 40.8 billion tons of CO2 equivalent was emitted when other greenhouse gases are also taken into account.
Too many countries are banking on carbon capture to reach net zero.
Problem: Over optimistic onshore wind and hydroelectric power
Solution: Climate change adjustments to projected capacity factors
Capacity refers to the maximum amount of energy that installations can produce, not what they are necessarily generating. The capacity factor is the total amount of electrical energy produced during a period of time divided by the amount of energy the plant could have produced at full capacity during that time period.
Due to changing global wind patterns many wind turbines are producing less electricity than projected.
For example, in 2021 there was less wind energy in the UK. India’s wind power generation was also down by around 40% during the peak wind season June- September which accounts for 3/4 of India’s annual production.
It takes a lot of time and resources to build all the new concrete foundations, roads, and power lines to the remote areas that have ample wind resources. What is left out of the discussion is that by the time of completion the amount of wind available at that site may be significantly less than planned, due to changing wind patterns associated with “climate change”, rendering many wind turbines stranded assets. This is less of a problem for offshore wind, where wind speeds and wind direction don’t change as often and thus provide more reliable power. However, creating and maintaining offshore wind infrastructure is expensive and complex.
Climate change is also resulting in changing global precipitation patterns.
In recent years the western United States has been suffering a megadrought and thus there has been a substantial decrease in hydroelectric energy.
Thus, for both wind and hydroelectric power a “climate change adjustment” needs to be made to the capacity factor to have a realistic idea of actual green energy that will be generated versus theoretical potential.
Problem: Carbon offsets
Solution: Continue planting trees but don’t grant carbon offsets for such efforts.
Carbon offsets based on planting trees should be banned.
The reason is that trees can no longer be counted on to sequester carbon. Increasingly, drought and forest fires are causing trees to add carbon dioxide to the atmosphere, not remove it, as demonstrated by the recent forest fires in Canada.
It would be sobering to keep track of all the carbon offsets based on tree planting which have gone up in smoke due to wildfires.
However, a baseline number of trees should be planted worldwide anyway because trees can be useful for things such as countering the urban heat island effect, preventing erosion and landslides, combating desertification, and sequestering carbon – at least temporarily.
The Wikipedia article “Carbon offset” lists many other types of carbon offsets besides planting trees that are more legitimate.
A typical tree can absorb around 21 kilograms of CO2 per year when fully grown – saplings absorb significantly less. Over a lifetime of 100 years, one tree can absorb 1 ton of CO2. This counts as one carbon credit.
Planting trees to hit global net zero carbon targets by 2050 with tree planting alone is mathematically impossible because it would require at least 1.6 billion hectares of new forest, equivalent to an area five times the size of India.
Problem: Traditional QE and Green QE
Solution: Environmental Easing
Given all the problems with traditional Quantitative Easing (QE) and Green QE a better alternative is needed. “Environmental Easing” is a mix of traditional QE and Green QE with significant differences from both.
Environmental Easing is exclusively focused on global warming and environmental degradation, which are existential threats to humanity.
Environmental Easing is a complicated and controversial topic which cannot be fully explored in this presentation. Please read the detailed discussion of Environmental Easing elsewhere on the HerculesGS website for an in depth analysis and exposition.
Problem: Fossil Peaker Power Plants
Solution: Iron-air batteries and Energy Vault gravity-based storage
Peakers are power plants that generally run only when there is a high demand, known as peak demand, for electricity. Recently peaker plants have been replaced with battery storage in some places. Even using Tesla Megapacks, battery storage can be 30% cheaper than gas peaker plants.
Most chemical batteries like Tesla’s lithium ion batteries last no more than 10 to 15 years and grid scale chemical batteries may last only 7 to 10 years.
The biggest problem with chemical batteries is that they require metals which are expensive and result in damage to the environment when they are mined. One promising exception is iron-air batteries developed by Form Energy. Made with the safest, cheapest, and most abundant materials on the planet: iron, water, and air they can store energy at 1/10 the cost of lithium-ion batteries.
Pumped-storage hydroelectricity is also sometimes used as a peaker where available. However, Energy Vault is a new technology that isn’t restricted by geography as hydroelectricity is.
Energy Vault offers gravity-based, grid scale energy storage with 80-90% round trip efficiency and no degradation in storage capacity over time.
Based on simple physics the system uses excess renewable energy to power electric motors that raise bricks weighing about 35 tons, then lowers the bricks to turn electric motors and generate electricity during peak demand – all controlled by AI software and executed autonomously.
At a cost of about $111 per megawatt hour (MWh) at present, dropping to $65 per MWh by 2025 Energy Vault is cost-efficient, reliable, safe to operate, and environmentally sustainable over a 35-year technical life.
Globally, the need for energy storage deployments is expected to grow to almost 830 gigawatt hour (GWh) by 2030. Energy Vault can meet a significant portion of this demand for long duration energy storage (i.e. 10 hours or more) by becoming as common as water towers in every town and city in the world.
Even if the entire amount of 830 GWh were met with Energy Vault structures it would only cost $54 million (65 X 1,000 = $65,000/GWh and 65,000 X 830 = $54 million).
In contrast Tesla Megapacks cost $327-412/KWh. Moreover annual maintenance cost for Megapacks starts at $6,570 and escalates at 2% per year. Due to inflation and supply chain issues there is also a significant backlog of orders for the Megapack.
Energy Vault structures can be built simultaneously across the world using locally available materials.
Despite the advantages of Energy Vault over chemical batteries, the world will need 85-140 terawatt hour (TWh) of long duration energy storage (>8 hours) by 2040 to store 10% of all electricity. The only other conceivable ways to store this much energy for extended periods of time are as hydrogen or ammonia.
Problem: Green hydrogen as fuel for cars and heating.
Solution: Closed loop hydrogen and ammonia for long duration and seasonal energy storage.
Green hydrogen refers to hydrogen that is made from the electrolysis of water using green electricity from wind and solar.
The use of green hydrogen as fuel for cars and for heating is very inefficient and requires a massive amount of investment in infrastructure and a continuous supply of fresh water.
Liquid hydrogen requires special thermally insulated containers and special handling. Even with thermally insulated containers the hydrogen will gradually leak away (usually at a rate of 1% per day).
For transportation, battery electric vehicles are much more efficient than fuel cell electric vehicles in terms of total energy use.
For heating, electric heat pumps are much more efficient than burning hydrogen. Heat pumps can deliver considerably more than one unit of heat (or cooling) per unit of electrical energy consumed.
Presently the most efficient residential heat pumps can deliver heat at 600% efficiency, compared with a gas heater at 50%-95% efficiency. When the heat is produced using renewable electricity, this delivers astonishing reductions in greenhouse gas emissions.
Moreover, natural gas pipelines and infrastructure need to be significantly upgraded or completely new pipelines need to be built in order to move gaseous hydrogen.
Closed-loop hydrogen is where excess renewable electricity is used to electrolyse water to produce hydrogen and oxygen, but the hydrogen would not be piped or liquified and transported to the point of use. The hydrogen would stay on site and be stored in large underground caverns for long duration and seasonal energy storage.
The hydrogen would be used to generate electricity when needed using fuel cells and the water generated would be collected and reused for electrolysis to generate hydrogen. The closed loop is water to hydrogen, back to water, back to hydrogen, …
Using HVDC transmission lines, electricity from utility-scale solar farms would go to geologically suitable hydrogen storage locations.
Salt caverns cost 10 times less than above ground tanks and 20 times less than hard rock mines. Caverns can be created in salt domes by drilling into the salt dome and injecting the rock with water, which dissolves the salt. The resulting brine is extracted, leaving a large cavity.
Green hydrogen can be stored in the salt dome and reconverted to electricity when needed and sent back to the grid. This is the only feasible method of long-duration energy storage which can shift excess energy from periods of oversupply, like California in the spring, to periods of undersupply, like California in the late summer.
Hydrogen can be produced with renewable energy from sources like solar panels and then stored underground in salt caverns for future use.
The Advanced Clean Energy Storage project in Utah aims to build the world’s largest storage facility for 1,000 megawatts of clean power using underground salt caverns to store hydrogen.
The salt dome formation has the potential to create up to 100 caverns, with each capable of holding 150 GWh of energy.
Most people are already familiar with the US Strategic Petroleum Reserve which holds oil in salt caverns in Louisiana and Texas. The Gulf Coast has sufficient salt dome potential to create a ‘Strategic Green Hydrogen Reserve’ larger than the one in Utah.
Parts of Europe and the Middle East also have significant salt dome potential for green hydrogen storage.
An ammonia economy uses ammonia as an alternative to gaseous hydrogen as an energy carrier by bonding hydrogen with nitrogen from the air to produce ammonia, which can be easily liquified, transported, and used (directly or indirectly) as a clean and renewable fuel. The advantages of ammonia are that it can be burned directly in an internal combustion engine with no carbon emissions or converted to electricity in a fuel cell.
Currently the Haber-Bosch (H-B) process used to produce ammonia from hydrogen typically operates at temperatures ranging from 325-525 C and high pressures ranging from 150-350 atmospheres in a reactor with an iron-based catalyst to produce ammonia at a yield of around 10-20%. These conditions have high-energy costs associated with them.
For some parts of the world, which do not have suitable geologic storage for hydrogen, liquid ammonia is a potential way to store large amounts of renewable energy for long periods of time.
A standard 60,000 m3 (cubic meters) tank of liquid ammonia contains about 211 GWh of energy, equivalent to the annual production of roughly 30 wind turbines.
Note that pumped-storage hydroelectricity (PSH) has a worldwide total installed storage capacity of 1.6 TWh, of which about 250 GWh are in the US. Thus, a single tank of ammonia contains nearly as much energy as all the pumped hydro in the US.
In 2021 in China, 13 new liquified natural gas (LNG) storage tanks that are part of five LNG terminal expansion projects entered into service, giving an additional 1.28 billion cubic meters of gas storage capacity. LNG tanks are much more difficult to manufacture than ammonia tanks. Furthermore, LNG tanks can also be used to store ammonia though not vice versa. Clearly, China has the resources to build sufficient green ammonia storage tanks to smooth out the power supply from solar and wind, or use existing LNG tanks to store the ammonia.
China has large sparsely populated regions where massive solar parks can be built with the sole purpose of generating green ammonia which can be used later to provide baseload power during cloudy days.
Note that China has done numerous feasibility tests for Carbon Capture and Storage (CCS) and it has been estimated that China has adequate deep geologic storage capacity for carbon dioxide storage to meet demand for more than 100 years.
However, the main obstacle is the cost which is $40-120 per ton of CO2. In 2020 China’s coal fired power plants emitted over 7.4 billion tons of CO2 which would cost $300-900 billion each year to sequester. For that much money China could easily build enough solar and wind projects and long duration energy storage to meet all its energy needs.
Problem: Rooftop solar
Solution: Utility-scale solar
Utility-scale solar is cheaper to install than rooftop solar given economies of scale and lower balance-of-system (BOS) costs (i.e. upfront costs with hard costs such as costs of inverters, switches, support racks, wiring, and batteries/storage systems and soft costs such as planning, permissions, customer acquisition, insurance and labor for installation and interconnection).
Many countries have arid land or deserts that serve no useful purpose. Given that wind and hydroelectric projects are problematic as discussed earlier, ‘Utility-scale solar’ is the best choice to generate green electricity. There is enough solar energy available in deserts and arid regions to provide all the world’s energy needs.
These solar plants can be built within a few years because they are built almost entirely with modular, readily available materials.
Note that on average it can take 10 years or more to build a high-voltage DC transmission (HVDC) line. However, the State Grid Corporation of China (SGCC) has built some Ultra HVDC (UHVDC) lines in Brazil in 2 years as part of China’s Belt and Road Initiative (BRI). Thus, an accelerated timeframe is possible.
Problem: Silicon Based Photovoltaic (PV) cells
Solution: CZTS thin film solar
Most commercial solar PV modules use photovoltaic cells (solar cells) made from highly purified silicon (Si).
Every step in their production requires an input of fossil fuels – as the carbon reductants needed for smelting silicon from quartz “ore”, to provide manufacturing process heat and power, and transportation.
The only “renewable” materials consumed are obtained by deforestation – by burning large areas of tropical rainforest for charcoal (another carbon reductant) and to provide the wood chips that are necessary for all silicon smelters to function.
Silicon smelters, polysilicon refineries, and crystal growers all require uninterrupted, 24/7 power that comes mostly from coal.
In 2012 IBM achieved a world-record PV solar-to-electric power conversion efficiency of 11.1 percent for thin-film CZTS (Copper, Zinc, Tin, Sulfide) which can be manufactured by simple ink-based techniques such as printing and casting.
CZTS uses only earth-abundant elements. So, even if thousands of GW are manufactured prices won’t skyrocket.
Estimates of global material reserves (for Cu, Zn, Sn, and S) suggest we could produce enough energy to power the world with only 0.1% of the available raw material resources.
CZTS PV cells could potentially yield up to 500 GW per year. Even if CZTS solar panels ultimately produce 40% less energy than the headline 500 GW they could still yield 300 GW of additional green energy to the world each year.
To put that in perspective, since solar cells were first invented in 1954 until today the installed base of photovoltaics just reached 1 terrawatt (TW).
The efficiency of CZTS panels is approximately a half to a third of silicon-based PV. Thus, 2 to 3 times the land area would be required to generate an equivalent amount of electricity.
However, since the solar farms are being located in deserts, arid regions, and/or agricultural land, space is not a limiting factor.
Thin-film solar panels have a better temperature coefficient than silicon-based panels. They are less affected by high temperatures and will lose only a small portion of their performance when it gets too hot. Thus, thin-film cells are recommended for use in deserts where there is plenty of sun and space.
Recycling thin film solar panels is also much easier than recycling silicon-based panels.
Moreover, the silicon (i.e. the most critical part) in the PV cell cannot be recycled. This means that replacing crystalline silicon-based panels with similar ones would have nearly the same detrimental impact on the environment as the original panels.
There are many different solar cell technologies in development, particularly third generation panels, that promise even higher efficiency than existing crystalline silicon. However, it would take years for them to be deployed outside the lab and scale economically.
Given that Environmental Easing will be deployed over decades, use of these new technologies at some point in the future isn’t ruled out. However, at present CZTS would be the one deployed first.
Problem: COP 26 Methane pledge
Solution:
Cut methane emissions by 75%
During COP 26 105 countries pledged to cut methane emissions by 30%. However, the largest emitters such as China, India, Russia, and Australia did not sign on. This is clearly inadequate.
More than 75% of methane emissions could be mitigated with existing technology today – and up to 40% at no net cost, according to the International Energy Association (IEA).
Methane is responsible for 25% of the global warming we are currently experiencing. Methane has global warming potential of more than 80 times that of CO2 during the 20 years following its release into the atmosphere.
China, India, Russia, and Australia as well as the rest of the world can easily use Environmental Easing to fund the relatively low-cost measures to cut methane emissions.
Problem: Climate change adaptation
Solution: Environmental Easing can be a lifeline for smaller countries
The Climate Adaptation Project List of the United Nations (UN) contains over 75 projects in over 50 countries.
However, in 2020 the total value of the adaptation project portfolio managed by UNEP’s Climate Change Adaptation Unit was a paltry $340 million.
Estimated adaptation costs in developing countries could reach $300 billion every year by 2030.
Right now, only 21 percent of climate finance provided by wealthier countries goes towards adaptation. For smaller countries that don’t emit much CO2, but still feel a disproportionate part of the pain caused by global warming, a larger percentage of their Environmental Easing can go toward adaptation.
However, for larger developing countries with higher emissions, priority would be given to cutting emissions. The reason is that if the world does not drastically cut emissions now the cost of adaptation in the future will go through the roof and adaptation may become impossible.
Conclusion
There is still a chance the world can avoid the cataclysmic global warming it is currently on track to experience. Through new funding mechanisms and targeted investment in a limited number of key technologies, Hercules can significantly mitigate global warming and avoid untold suffering and trillions of dollars in damages from natural disasters and extreme weather.
References
https://www.cnbc.com/2015/08/18/cost-of-not-acting-on-climate-change-44-trillion-citi.html
https://www.oxfam.org/en/press-releases/net-zero-carbon-targets-are-dangerous-distractions-priority-cutting-emissions-says
https://www.washingtonpost.com/climate-environment/interactive/2021/greenhouse-gas-emissions-pledges-data/
https://en.wikipedia.org/wiki/Capacity_factor
https://www.livemint.com/industry/energy/india-wind-power-generation-down-40-during-peak-season-11601541656859.html
https://www.imf.org/external/pubs/ft/fandd/2021/06/the-future-of-emerging-markets-duttagupta-and-pazarbasioglu.htm
https://www.imf.org/Publications/fandd/issues/2021/09/isabel-schnabel-ECB-climate-change
https://www.greencarcongress.com/2021/11/20211124-popovich.html
https://en.wikipedia.org/wiki/Efficient_energy_use
https://en.wikipedia.org/wiki/Greenhouse_gas_emissions_by_China
https://www.bbc.com/future/article/20211028-how-chinas-climate-decisions-affect-the-world
https://helena.org/projects/energy-vault
https://www.businesswire.com/news/home/20211123005581/en/New-LDES-Council-Report-Finds-Up-to-140-TWh-of-Long-Duration-Energy-Storage-Needed-to-Enable-Grid-Net-Zero-by-2040-at-Lowest-Cost
https://www.cnbc.com/2020/11/01/how-salt-caverns-may-trigger-11-trillion-hydrogen-energy-boom-.html
https://en.wikipedia.org/wiki/Grid_energy_storage
https://en.wikipedia.org/wiki/Pumped-storage_hydroelectricity
https://en.wikipedia.org/wiki/Carbon_offset
http://global.chinadaily.com.cn/a/202112/29/WS61cbc19aa310cdd39bc7e0c6.html
https://phys.org/news/2009-10-carbon-capture-major-potential-china.html
https://www.power-technology.com/features/featurethe-worlds-longest-power-transmission-lines-4167964/
https://www.researchgate.net/publication/335083312_Why_do_we_burn_coal_and_trees_to_make_solar_panels
https://www.ibm.com/blogs/research/2012/08/shedding-light-on-new-frontiers-of-solar-cell-semiconductors/
https://en.wikipedia.org/wiki/CZTS
https://www.researchgate.net/publication/348493217_Analysis_of_manufacturing_cost_and_market_niches_for_Cu2ZnSnS4_CZTS_solar_cells
https://www.pv-magazine.com/2022/03/15/humans-have-installed-1-terawatt-of-solar-capacity/#:~:text=The%20world%20has%20very%20recently,at%20the%20end%20of%202020.
https://www.newscientist.com/article/2295810-cop26-105-countries-pledge-to-cut-methane-emissions-by-30-per-cent/
https://www.iea.org/reports/methane-tracker-2020
https://www.edf.org/climate/methane-crucial-opportunity-climate-fight
https://www.unep.org/explore-topics/climate-action/what-we-do/climate-adaptation/access-adaptation-finance#:~:text=In%202020%2C%20the%20total%20value,%2440%20million%20in%20bilateral%20funds.
Hercules Global Solutions
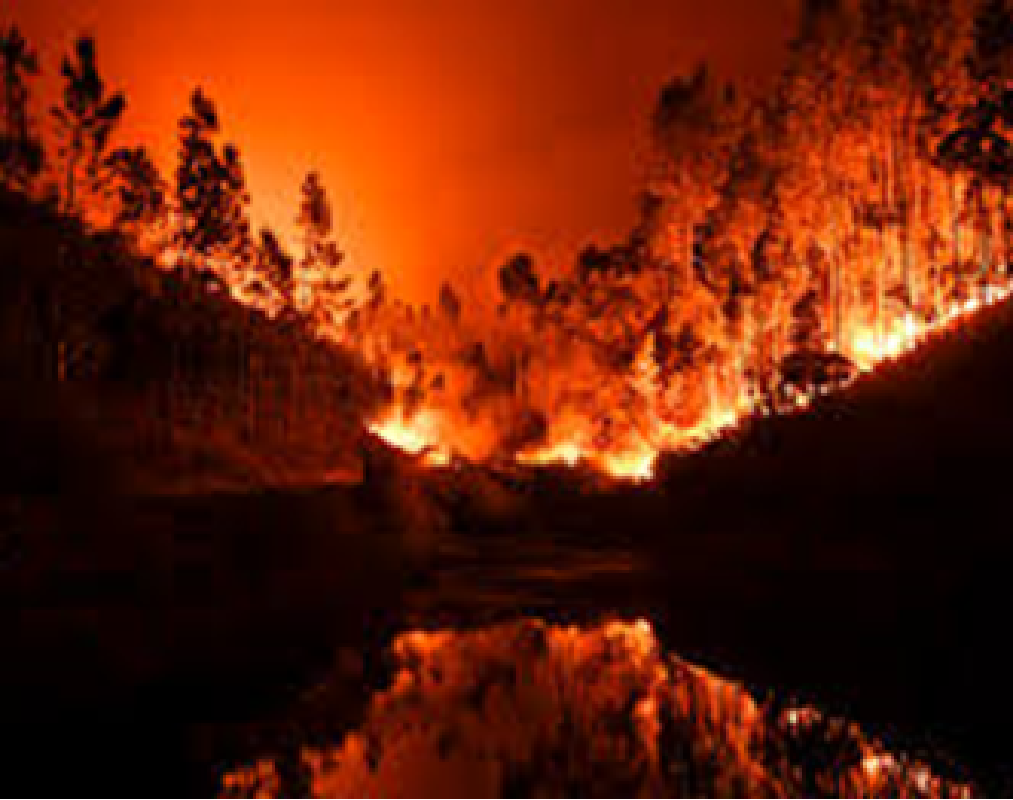
The last seven years were the world’s hottest years on record since record-keeping began in 1880
Unless drastic action is taken soon the world will increasingly suffer from more intense storms, fires, heatwaves, famines, and floods
Banking giant Citigroup estimates that unchecked climate change will cost $44 Trillion by 2060
This presentation discusses the biggest problems related to global warming and suggests unconventional solutions to address them.
The goal of this presentation is to provide a roadmap for the world to significantly reduce global warming.
Table of Contents
Problem: Net zero
Solution: Real Zero
Problem: Over optimistic onshore wind and hydroelectric power
Solution: Climate change adjustments to projected capacity factors
Problem: Carbon offsets
Solution: Continue planting trees but don’t grant carbon offsets for such efforts.
Problem: Traditional QE and Green QE
Solution: Environmental Easing
Problem: Fossil Peaker Power Plants
Solution: Iron-air batteries and Energy Vault gravity-based storage
Problem: Green hydrogen as fuel for cars and heating.
Solution: Closed loop hydrogen and ammonia for long duration and seasonal energy storage.
Problem: Rooftop solar
Solution: Utility-scale solar
Problem: Silicon Based Photovoltaic (PV) cells
Solution: CZTS thin film solar
Problem: COP 26 Methane pledge
Solution: Cut methane emissions by 75%
Problem: Climate change adaptation
Solution: Environmental Easing can be a lifeline for smaller countries
Conclusion
References
Novel Solutions
Problem: Net zero
Solution: Real Zero
As a new Oxfam report stated, ‘Net zero’ carbon targets are dangerous distractions from the priority of cutting emissions. Rich nations and corporates are continuing “dirty business as usual”. Most of their pledges are vague and not backed by measurable plans.
Removing emissions is not a substitute for cutting emissions, and these should be counted separately.
As it is most countries cannot be trusted to follow through on their promises. As the Washington Post recently reported many countries under report their greenhouse gas emissions to the United Nations, in aggregate by anywhere from 8.5 to 13.3 billion tons per year.
Rather than celebrating and letting a country off the hook whenever they say the magical words ‘net zero’ and some far-off date in the future when they will achieve it, it would be helpful instead to assess each country’s commitments and actions and grade them from A to F on how much they are actually decarbonizing.
A simple decarbonization table for each country would show each sector of its economy, what emissions were, what they should be, and how it plans to decarbonize further.
Going forward the goal should be ‘Real zero’ instead of ‘Net zero’. Real zero means zero emissions of carbon. Only with this as the goal will the world be on a credible path to Net Zero. Fanciful plans for carbon removal, such as with Direct Air Capture (DAC) should not allow countries to skip the hard work of decarbonizing.
For an 83% chance of limiting global warming to 1.5 C the world can only emit another 300 Gt CO2 (Gigatons, i.e. billion tons). In 2021 alone the world emitted over 36 billion tons of CO2. 40.8 billion tons of CO2 equivalent was emitted when other greenhouse gases are also taken into account.
Too many countries are banking on carbon capture to reach net zero.
Problem: Over optimistic onshore wind and hydroelectric power
Solution: Climate change adjustments to projected capacity factors
Capacity refers to the maximum amount of energy that installations can produce, not what they are necessarily generating. The capacity factor is the total amount of electrical energy produced during a period of time divided by the amount of energy the plant could have produced at full capacity during that time period.
Due to changing global wind patterns many wind turbines are producing less electricity than projected.
For example, in 2021 there was less wind energy in the UK. India’s wind power generation was also down by around 40% during the peak wind season June- September which accounts for 3/4 of India’s annual production.
It takes a lot of time and resources to build all the new concrete foundations, roads, and power lines to the remote areas that have ample wind resources. What is left out of the discussion is that by the time of completion the amount of wind available at that site may be significantly less than planned, due to changing wind patterns associated with “climate change”, rendering many wind turbines stranded assets. This is less of a problem for offshore wind, where wind speeds and wind direction don’t change as often and thus provide more reliable power. However, creating and maintaining offshore wind infrastructure is expensive and complex.
Climate change is also resulting in changing global precipitation patterns.
In recent years the western United States has been suffering a megadrought and thus there has been a substantial decrease in hydroelectric energy.
Thus, for both wind and hydroelectric power a “climate change adjustment” needs to be made to the capacity factor to have a realistic idea of actual green energy that will be generated versus theoretical potential.
Problem: Carbon offsets
Solution: Continue planting trees but don’t grant carbon offsets for such efforts.
Carbon offsets based on planting trees should be banned.
The reason is that trees can no longer be counted on to sequester carbon. Increasingly, drought and forest fires are causing trees to add carbon dioxide to the atmosphere, not remove it, as demonstrated by the recent forest fires in Canada.
It would be sobering to keep track of all the carbon offsets based on tree planting which have gone up in smoke due to wildfires.
However, a baseline number of trees should be planted worldwide anyway because trees can be useful for things such as countering the urban heat island effect, preventing erosion and landslides, combating desertification, and sequestering carbon – at least temporarily.
The Wikipedia article “Carbon offset” lists many other types of carbon offsets besides planting trees that are more legitimate.
A typical tree can absorb around 21 kilograms of CO2 per year when fully grown – saplings absorb significantly less. Over a lifetime of 100 years, one tree can absorb 1 ton of CO2. This counts as one carbon credit.
Planting trees to hit global net zero carbon targets by 2050 with tree planting alone is mathematically impossible because it would require at least 1.6 billion hectares of new forest, equivalent to an area five times the size of India.
Problem: Traditional QE and Green QE
Solution: Environmental Easing
Given all the problems with traditional Quantitative Easing (QE) and Green QE a better alternative is needed. “Environmental Easing” is a mix of traditional QE and Green QE with significant differences from both.
Environmental Easing is exclusively focused on global warming and environmental degradation, which are existential threats to humanity.
Environmental Easing is a complicated and controversial topic which cannot be fully explored in this presentation. Please read the detailed discussion of Environmental Easing elsewhere on the HerculesGS website for an in depth analysis and exposition.
Problem: Fossil Peaker Power Plants
Solution: Iron-air batteries and Energy Vault gravity-based storage
Peakers are power plants that generally run only when there is a high demand, known as peak demand, for electricity. Recently peaker plants have been replaced with battery storage in some places. Even using Tesla Megapacks, battery storage can be 30% cheaper than gas peaker plants.
Most chemical batteries like Tesla’s lithium ion batteries last no more than 10 to 15 years and grid scale chemical batteries may last only 7 to 10 years.
The biggest problem with chemical batteries is that they require metals which are expensive and result in damage to the environment when they are mined. One promising exception is iron-air batteries developed by Form Energy. Made with the safest, cheapest, and most abundant materials on the planet: iron, water, and air they can store energy at 1/10 the cost of lithium-ion batteries.
Pumped-storage hydroelectricity is also sometimes used as a peaker where available. However, Energy Vault is a new technology that isn’t restricted by geography as hydroelectricity is.
Energy Vault offers gravity-based, grid scale energy storage with 80-90% round trip efficiency and no degradation in storage capacity over time.
Based on simple physics the system uses excess renewable energy to power electric motors that raise bricks weighing about 35 tons, then lowers the bricks to turn electric motors and generate electricity during peak demand – all controlled by AI software and executed autonomously.
At a cost of about $111 per megawatt hour (MWh) at present, dropping to $65 per MWh by 2025 Energy Vault is cost-efficient, reliable, safe to operate, and environmentally sustainable over a 35-year technical life.
Globally, the need for energy storage deployments is expected to grow to almost 830 gigawatt hour (GWh) by 2030. Energy Vault can meet a significant portion of this demand for long duration energy storage (i.e. 10 hours or more) by becoming as common as water towers in every town and city in the world.
Even if the entire amount of 830 GWh were met with Energy Vault structures it would only cost $54 million (65 X 1,000 = $65,000/GWh and 65,000 X 830 = $54 million).
In contrast Tesla Megapacks cost $327-412/KWh. Moreover annual maintenance cost for Megapacks starts at $6,570 and escalates at 2% per year. Due to inflation and supply chain issues there is also a significant backlog of orders for the Megapack.
Energy Vault structures can be built simultaneously across the world using locally available materials.
Despite the advantages of Energy Vault over chemical batteries, the world will need 85-140 terawatt hour (TWh) of long duration energy storage (>8 hours) by 2040 to store 10% of all electricity. The only other conceivable ways to store this much energy for extended periods of time are as hydrogen or ammonia.
Problem: Green hydrogen as fuel for cars and heating.
Solution: Closed loop hydrogen and ammonia for long duration and seasonal energy storage.
Green hydrogen refers to hydrogen that is made from the electrolysis of water using green electricity from wind and solar.
The use of green hydrogen as fuel for cars and for heating is very inefficient and requires a massive amount of investment in infrastructure and a continuous supply of fresh water.
Liquid hydrogen requires special thermally insulated containers and special handling. Even with thermally insulated containers the hydrogen will gradually leak away (usually at a rate of 1% per day).
For transportation, battery electric vehicles are much more efficient than fuel cell electric vehicles in terms of total energy use.
For heating, electric heat pumps are much more efficient than burning hydrogen. Heat pumps can deliver considerably more than one unit of heat (or cooling) per unit of electrical energy consumed.
Presently the most efficient residential heat pumps can deliver heat at 600% efficiency, compared with a gas heater at 50%-95% efficiency. When the heat is produced using renewable electricity, this delivers astonishing reductions in greenhouse gas emissions.
Moreover, natural gas pipelines and infrastructure need to be significantly upgraded or completely new pipelines need to be built in order to move gaseous hydrogen.
Closed-loop hydrogen is where excess renewable electricity is used to electrolyse water to produce hydrogen and oxygen, but the hydrogen would not be piped or liquified and transported to the point of use. The hydrogen would stay on site and be stored in large underground caverns for long duration and seasonal energy storage.
The hydrogen would be used to generate electricity when needed using fuel cells and the water generated would be collected and reused for electrolysis to generate hydrogen. The closed loop is water to hydrogen, back to water, back to hydrogen, …
Using HVDC transmission lines, electricity from utility-scale solar farms would go to geologically suitable hydrogen storage locations.
Salt caverns cost 10 times less than above ground tanks and 20 times less than hard rock mines. Caverns can be created in salt domes by drilling into the salt dome and injecting the rock with water, which dissolves the salt. The resulting brine is extracted, leaving a large cavity.
Green hydrogen can be stored in the salt dome and reconverted to electricity when needed and sent back to the grid. This is the only feasible method of long-duration energy storage which can shift excess energy from periods of oversupply, like California in the spring, to periods of undersupply, like California in the late summer.
Hydrogen can be produced with renewable energy from sources like solar panels and then stored underground in salt caverns for future use.
The Advanced Clean Energy Storage project in Utah aims to build the world’s largest storage facility for 1,000 megawatts of clean power using underground salt caverns to store hydrogen.
The salt dome formation has the potential to create up to 100 caverns, with each capable of holding 150 GWh of energy.
Most people are already familiar with the US Strategic Petroleum Reserve which holds oil in salt caverns in Louisiana and Texas. The Gulf Coast has sufficient salt dome potential to create a ‘Strategic Green Hydrogen Reserve’ larger than the one in Utah.
Parts of Europe and the Middle East also have significant salt dome potential for green hydrogen storage.
An ammonia economy uses ammonia as an alternative to gaseous hydrogen as an energy carrier by bonding hydrogen with nitrogen from the air to produce ammonia, which can be easily liquified, transported, and used (directly or indirectly) as a clean and renewable fuel. The advantages of ammonia are that it can be burned directly in an internal combustion engine with no carbon emissions or converted to electricity in a fuel cell.
Currently the Haber-Bosch (H-B) process used to produce ammonia from hydrogen typically operates at temperatures ranging from 325-525 C and high pressures ranging from 150-350 atmospheres in a reactor with an iron-based catalyst to produce ammonia at a yield of around 10-20%. These conditions have high-energy costs associated with them.
For some parts of the world, which do not have suitable geologic storage for hydrogen, liquid ammonia is a potential way to store large amounts of renewable energy for long periods of time.
A standard 60,000 m3 (cubic meters) tank of liquid ammonia contains about 211 GWh of energy, equivalent to the annual production of roughly 30 wind turbines.
Note that pumped-storage hydroelectricity (PSH) has a worldwide total installed storage capacity of 1.6 TWh, of which about 250 GWh are in the US. Thus, a single tank of ammonia contains nearly as much energy as all the pumped hydro in the US.
In 2021 in China, 13 new liquified natural gas (LNG) storage tanks that are part of five LNG terminal expansion projects entered into service, giving an additional 1.28 billion cubic meters of gas storage capacity. LNG tanks are much more difficult to manufacture than ammonia tanks. Furthermore, LNG tanks can also be used to store ammonia though not vice versa. Clearly, China has the resources to build sufficient green ammonia storage tanks to smooth out the power supply from solar and wind, or use existing LNG tanks to store the ammonia.
China has large sparsely populated regions where massive solar parks can be built with the sole purpose of generating green ammonia which can be used later to provide baseload power during cloudy days.
Note that China has done numerous feasibility tests for Carbon Capture and Storage (CCS) and it has been estimated that China has adequate deep geologic storage capacity for carbon dioxide storage to meet demand for more than 100 years.
However, the main obstacle is the cost which is $40-120 per ton of CO2. In 2020 China’s coal fired power plants emitted over 7.4 billion tons of CO2 which would cost $300-900 billion each year to sequester. For that much money China could easily build enough solar and wind projects and long duration energy storage to meet all its energy needs.
Problem: Rooftop solar
Solution: Utility-scale solar
Utility-scale solar is cheaper to install than rooftop solar given economies of scale and lower balance-of-system (BOS) costs (i.e. upfront costs with hard costs such as costs of inverters, switches, support racks, wiring, and batteries/storage systems and soft costs such as planning, permissions, customer acquisition, insurance and labor for installation and interconnection).
Many countries have arid land or deserts that serve no useful purpose. Given that wind and hydroelectric projects are problematic as discussed earlier, ‘Utility-scale solar’ is the best choice to generate green electricity. There is enough solar energy available in deserts and arid regions to provide all the world’s energy needs.
These solar plants can be built within a few years because they are built almost entirely with modular, readily available materials.
Note that on average it can take 10 years or more to build a high-voltage DC transmission (HVDC) line. However, the State Grid Corporation of China (SGCC) has built some Ultra HVDC (UHVDC) lines in Brazil in 2 years as part of China’s Belt and Road Initiative (BRI). Thus, an accelerated timeframe is possible.
Problem: Silicon Based Photovoltaic (PV) cells
Solution: CZTS thin film solar
Most commercial solar PV modules use photovoltaic cells (solar cells) made from highly purified silicon (Si).
Every step in their production requires an input of fossil fuels – as the carbon reductants needed for smelting silicon from quartz “ore”, to provide manufacturing process heat and power, and transportation.
The only “renewable” materials consumed are obtained by deforestation – by burning large areas of tropical rainforest for charcoal (another carbon reductant) and to provide the wood chips that are necessary for all silicon smelters to function.
Silicon smelters, polysilicon refineries, and crystal growers all require uninterrupted, 24/7 power that comes mostly from coal.
In 2012 IBM achieved a world-record PV solar-to-electric power conversion efficiency of 11.1 percent for thin-film CZTS (Copper, Zinc, Tin, Sulfide) which can be manufactured by simple ink-based techniques such as printing and casting.
CZTS uses only earth-abundant elements. So, even if thousands of GW are manufactured prices won’t skyrocket.
Estimates of global material reserves (for Cu, Zn, Sn, and S) suggest we could produce enough energy to power the world with only 0.1% of the available raw material resources.
CZTS PV cells could potentially yield up to 500 GW per year. Even if CZTS solar panels ultimately produce 40% less energy than the headline 500 GW they could still yield 300 GW of additional green energy to the world each year.
To put that in perspective, since solar cells were first invented in 1954 until today the installed base of photovoltaics just reached 1 terrawatt (TW).
The efficiency of CZTS panels is approximately a half to a third of silicon-based PV. Thus, 2 to 3 times the land area would be required to generate an equivalent amount of electricity.
However, since the solar farms are being located in deserts, arid regions, and/or agricultural land, space is not a limiting factor.
Thin-film solar panels have a better temperature coefficient than silicon-based panels. They are less affected by high temperatures and will lose only a small portion of their performance when it gets too hot. Thus, thin-film cells are recommended for use in deserts where there is plenty of sun and space.
Recycling thin film solar panels is also much easier than recycling silicon-based panels.
Moreover, the silicon (i.e. the most critical part) in the PV cell cannot be recycled. This means that replacing crystalline silicon-based panels with similar ones would have nearly the same detrimental impact on the environment as the original panels.
There are many different solar cell technologies in development, particularly third generation panels, that promise even higher efficiency than existing crystalline silicon. However, it would take years for them to be deployed outside the lab and scale economically.
Given that Environmental Easing will be deployed over decades, use of these new technologies at some point in the future isn’t ruled out. However, at present CZTS would be the one deployed first.
Problem: COP 26 Methane pledge
Solution:
Cut methane emissions by 75%
During COP 26 105 countries pledged to cut methane emissions by 30%. However, the largest emitters such as China, India, Russia, and Australia did not sign on. This is clearly inadequate.
More than 75% of methane emissions could be mitigated with existing technology today – and up to 40% at no net cost, according to the International Energy Association (IEA).
Methane is responsible for 25% of the global warming we are currently experiencing. Methane has global warming potential of more than 80 times that of CO2 during the 20 years following its release into the atmosphere.
China, India, Russia, and Australia as well as the rest of the world can easily use Environmental Easing to fund the relatively low-cost measures to cut methane emissions.
Problem: Climate change adaptation
Solution: Environmental Easing can be a lifeline for smaller countries
The Climate Adaptation Project List of the United Nations (UN) contains over 75 projects in over 50 countries.
However, in 2020 the total value of the adaptation project portfolio managed by UNEP’s Climate Change Adaptation Unit was a paltry $340 million.
Estimated adaptation costs in developing countries could reach $300 billion every year by 2030.
Right now, only 21 percent of climate finance provided by wealthier countries goes towards adaptation. For smaller countries that don’t emit much CO2, but still feel a disproportionate part of the pain caused by global warming, a larger percentage of their Environmental Easing can go toward adaptation.
However, for larger developing countries with higher emissions, priority would be given to cutting emissions. The reason is that if the world does not drastically cut emissions now the cost of adaptation in the future will go through the roof and adaptation may become impossible.
Conclusion
There is still a chance the world can avoid the cataclysmic global warming it is currently on track to experience. Through new funding mechanisms and targeted investment in a limited number of key technologies, Hercules can significantly mitigate global warming and avoid untold suffering and trillions of dollars in damages from natural disasters and extreme weather.
References
https://www.cnbc.com/2015/08/18/cost-of-not-acting-on-climate-change-44-trillion-citi.html
https://www.oxfam.org/en/press-releases/net-zero-carbon-targets-are-dangerous-distractions-priority-cutting-emissions-says
https://www.washingtonpost.com/climate-environment/interactive/2021/greenhouse-gas-emissions-pledges-data/
https://en.wikipedia.org/wiki/Capacity_factor
https://www.livemint.com/industry/energy/india-wind-power-generation-down-40-during-peak-season-11601541656859.html
https://www.imf.org/external/pubs/ft/fandd/2021/06/the-future-of-emerging-markets-duttagupta-and-pazarbasioglu.htm
https://www.imf.org/Publications/fandd/issues/2021/09/isabel-schnabel-ECB-climate-change
https://www.greencarcongress.com/2021/11/20211124-popovich.html
https://en.wikipedia.org/wiki/Efficient_energy_use
https://en.wikipedia.org/wiki/Greenhouse_gas_emissions_by_China
https://www.bbc.com/future/article/20211028-how-chinas-climate-decisions-affect-the-world
https://helena.org/projects/energy-vault
https://www.businesswire.com/news/home/20211123005581/en/New-LDES-Council-Report-Finds-Up-to-140-TWh-of-Long-Duration-Energy-Storage-Needed-to-Enable-Grid-Net-Zero-by-2040-at-Lowest-Cost
https://www.cnbc.com/2020/11/01/how-salt-caverns-may-trigger-11-trillion-hydrogen-energy-boom-.html
https://en.wikipedia.org/wiki/Grid_energy_storage
https://en.wikipedia.org/wiki/Pumped-storage_hydroelectricity
https://en.wikipedia.org/wiki/Carbon_offset
http://global.chinadaily.com.cn/a/202112/29/WS61cbc19aa310cdd39bc7e0c6.html
https://phys.org/news/2009-10-carbon-capture-major-potential-china.html
https://www.power-technology.com/features/featurethe-worlds-longest-power-transmission-lines-4167964/
https://www.researchgate.net/publication/335083312_Why_do_we_burn_coal_and_trees_to_make_solar_panels
https://www.ibm.com/blogs/research/2012/08/shedding-light-on-new-frontiers-of-solar-cell-semiconductors/
https://en.wikipedia.org/wiki/CZTS
https://www.researchgate.net/publication/348493217_Analysis_of_manufacturing_cost_and_market_niches_for_Cu2ZnSnS4_CZTS_solar_cells
https://www.pv-magazine.com/2022/03/15/humans-have-installed-1-terawatt-of-solar-capacity/#:~:text=The%20world%20has%20very%20recently,at%20the%20end%20of%202020.
https://www.newscientist.com/article/2295810-cop26-105-countries-pledge-to-cut-methane-emissions-by-30-per-cent/
https://www.iea.org/reports/methane-tracker-2020
https://www.edf.org/climate/methane-crucial-opportunity-climate-fight
https://www.unep.org/explore-topics/climate-action/what-we-do/climate-adaptation/access-adaptation-finance#:~:text=In%202020%2C%20the%20total%20value,%2440%20million%20in%20bilateral%20funds.